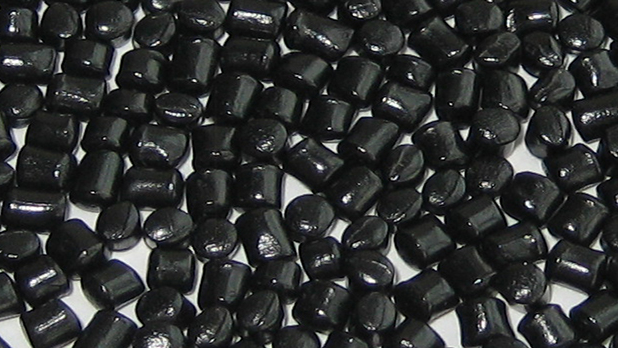
Researchers are delving into the potential of hard carbon anodes as a sustainable, high-capacity replacement for conventional graphite anodes in sodium-ion, lithium-ion, and potassium-ion batteries. A team from Imperial College London published about this in Advanced Materials. Hard carbon demonstrates a high ion storage capacity, sustainability, and a wide availability of raw materials. The study reveals that the electrochemical performance of these anodes is significantly influenced by the pyrolysis temperature, drastically affecting their structure. Hard carbon anodes not only excel in lithium-ion batteries due to their high surface area and defect-rich structure, but also in sodium-ion batteries, where they display superior performance at lower current densities. This research highlights the potential of hard carbon anodes for propelling next-generation battery technologies beyond traditional lithium-ion batteries.
- The use of hard carbon anodes in sodium-ion, lithium-ion, and potassium-ion batteries, revealing structural variations that impact their electrochemical performance.
- Lower-temperature synthesized hard carbon (G1000) excels in lithium-ion batteries due to its high surface area and defect-rich structure, while G1500 demonstrates superior performance in sodium-ion batteries, offering a balance of defects, interlayer spacing, and porosity.
- Hard carbon’s potential advantages, including sustainability, high capacity, high power density, and long-term stability, position it as a promising candidate for advancing next-generation battery technologies.
Unlocking the potential of hard carbon anodes
At the heart of the research is a deep exploration of hard carbon, a material rich in promise for future battery systems. Hard carbon, as its name suggests, is a form of carbon that is structurally denser than other forms. Its high ion storage capacity makes it an attractive candidate for the anodes in battery systems. Anodes, a key component of batteries, are where the ions are stored before they travel through the electrolyte to the cathode, producing an electric current.
What sets hard carbon apart from other anode materials, such as the commonly used graphite, is its structural versatility. The structure of hard carbon can be manipulated through the process of hydrothermal carbonisation and pyrolysis, the methods used to synthesise it.
Understanding hydrothermal carbonisation and pyrolysis
Hydrothermal carbonisation is a process that involves the decomposition of organic material at high pressure and a temperature below its carbonisation point, typically in the range of 180-250 degrees Celsius. This process leads to the formation of a highly carbonaceous solid known as hydrochar. Pyrolysis, on the other hand, involves heating the hydrochar in the absence of oxygen, causing the material to undergo physical and chemical changes. During pyrolysis, volatile substances are removed, leaving behind the carbon-rich hard carbon.
The pyrolysis temperature is a critical factor in determining the characteristics of the hard carbon. As the temperature increases, so does the structural order of the hard carbon, which influences the interlayer distance, number of layers, porosity, and surface functional groups. These properties, in turn, determine the performance of the hard carbon as an anode in different battery systems.
Performance in various battery systems
In lithium-ion batteries (LIBs), hard carbon synthesised at lower temperatures—designated as G1000—demonstrated superior performance. This is attributed to its high surface area and defect-rich structure, which enhances the storage capacity of lithium ions. This is a major advantage, as the capacity of a battery is directly tied to the number of ions it can store.
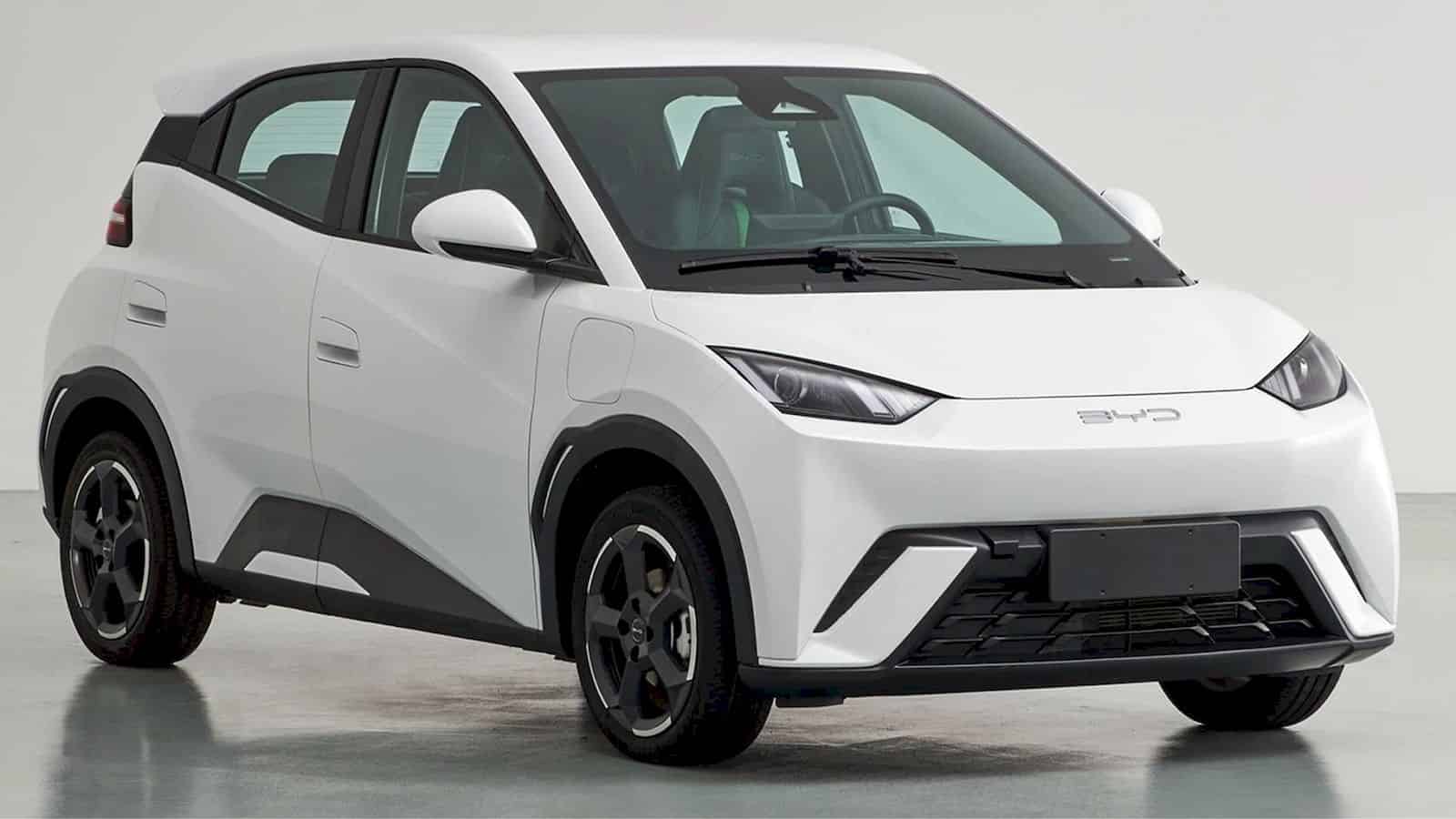
However, when it comes to sodium-ion batteries (NIBs), the performance dynamics change. Here, hard carbon synthesised at a higher temperature—known as G1500—outperforms its lower-temperature counterpart at lower current densities. The superior performance of G1500 can be traced back to its balance of defects, interlayer spacing, and porosity. These characteristics make G1500 better equipped to store sodium ions, which are larger than lithium ions.
What the future holds for hard carbon anodes
Given these results, it’s clear to see why hard carbon anodes are garnering attention. They offer a path to more sustainable, high-capacity batteries, with the potential to push the boundaries of current lithium-ion battery technology. Not only do they show promise in both lithium-ion and sodium-ion batteries, but they can also be used in potassium-ion batteries (KIBs), thereby diversifying their applications.
Moreover, hard carbon is abundant and sustainable. It can be derived from a wide range of sources, making it a more environmentally friendly alternative. The high ion storage capacity of hard carbon could also lead to batteries with higher energy densities, making them suitable for applications requiring rapid energy discharge.