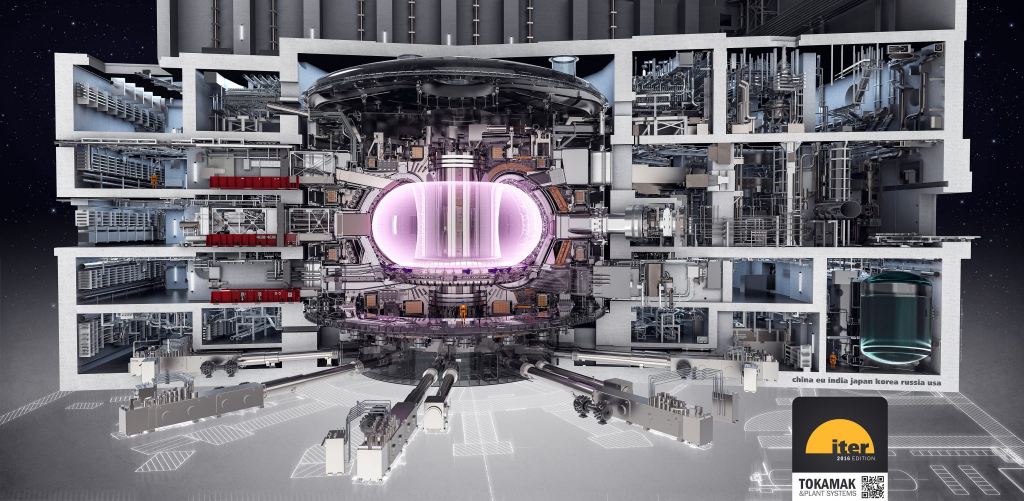
The most important topic these days is energy. Not only because there is a crisis in that area due to the war between Russia and Ukraine. If we want to avoid the consequences of far-reaching climate change, we will have to manage without fossil fuels. One of the solutions that – after years of being maligned – is back on the agenda is nuclear energy. For decades, scientists have been working to develop alternatives to nuclear energy that are safer and produce more energy than today’s nuclear fission.
What nuclear energy alternatives exist?
Some highly discussed alternatives are:
- Generation IV-reactors – A group of six nuclear reactors developed with the aim to be sustainable, safe, reliable, efficient, and have a lower operational cost than conventional nuclear fission reactors.
- Nuclear fusion – The process that also takes place in the sun. The fusion of two atoms releases energy. There is hardly any radiation waste.
Why should we use nuclear power?
Nuclear plants are considered an important tool for reducing our CO2 emissions.
How large will the alternative nuclear plants be?
It depends. Current nuclear fusion reactors are quite large so that the plasma temperature does not decrease easily. Generation IV reactors such as molten salt reactors are small so that they can be assembled inside a factory and shipped to another location.
Is it realistic that any of these alternatives will operate commercially?
Yes. In fact, some already are.
Controversial, but necessary
Few energy sources are as controversial as nuclear energy. Mostly because of the nuclear disasters in Fukushima and Chernobyl. On the other hand, researchers believe it is the only solution to tackle climate change. “Nuclear energy is an indispensable tool for achieving the global sustainable development agenda,” writes the United Nations Economic Commission for Europe in its report on nuclear energy.
“It’s important to note that today’s reactors are not unsafe or poorly designed. It’s just that the fourth generation reactors are even better,” says professor Jan Emblemsvåg at the Norwegian University for Science and Technology.
Safer, more sustainable fission reactors
Generation IV consists of six reactors aimed at being more economically and energetically efficient, safer, and more sustainable than current light water reactors. Each has its own distinct characteristics, but all are run on the same fissile fuel that is used today, such as uranium. Except for one, which can run both on uranium and on other less-radioactive material such as thorium. This is the molten salt-reactor (MSR).
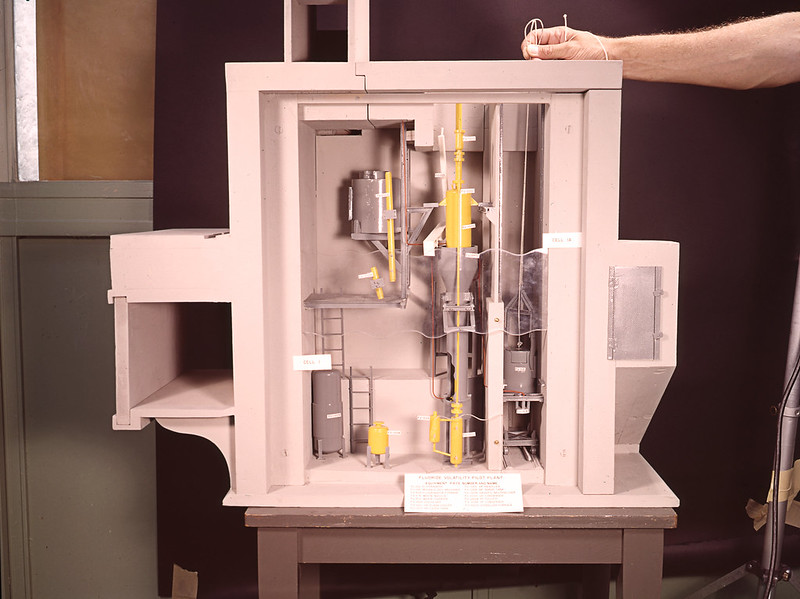
“In many ways, you could compare it to a stew. Imagine a stew simmering on the stove. When the fuel and coolant is integrated into one medium, the reactor becomes much less complex design-wise,” says Emblemsvåg about the molten salt reactor. In a conventional light-water reactor, uranium is typically used as fuel, while water works as both a coolant and a neutron moderator slowing down the speed of neutrons in the reactor. To increase the thermal effectivity, the water is held in high pressure. In a MSR the coolant – the salt – is also the fuel.
The salt makes MSRs able to use non-fissile elements, such as thorium which is fertile instead of fissile. In an MSR, thorium can be dissolved with the salt and converted to the radioactive uranium-233. This is beneficial because the radioactive levels of thorium are too low to cause any harm on its own. “You could walk around with thorium in the pockets of your pants your entire life,” says Emblemsvåg.
Thorium is also abundant and can be found in various places on Earth. This is good news geopolitically speaking, as it becomes easier to switch supply chains should one country stop extracting or exporting thorium. In comparison, around 40 percent of all uranium is found in China, which means the country has a great stronghold on the uranium market.
Developed in the 1960s
MSR is not a new technology. In the 1960s, Oak Ridge National Laboratory in the US was researching MSR alongside other nuclear energy sources. Already then, researchers knew that MSRs were a realistic and important source for energy in the future. However, research took an abrupt turn in the mid-1960s when the US Navy needed quick access to atomic energy. Light-water reactors were the faster option, and so they were used and MSR was forgotten.
In the 1980s-1990s, little to no research on MSR was conducted, except in Japan where some research was conducted under the Fuji-reactor umbrella. It did not return until after 9/11, when US authorities began to fear that radioactive rest material could be used to create dirty bombs.
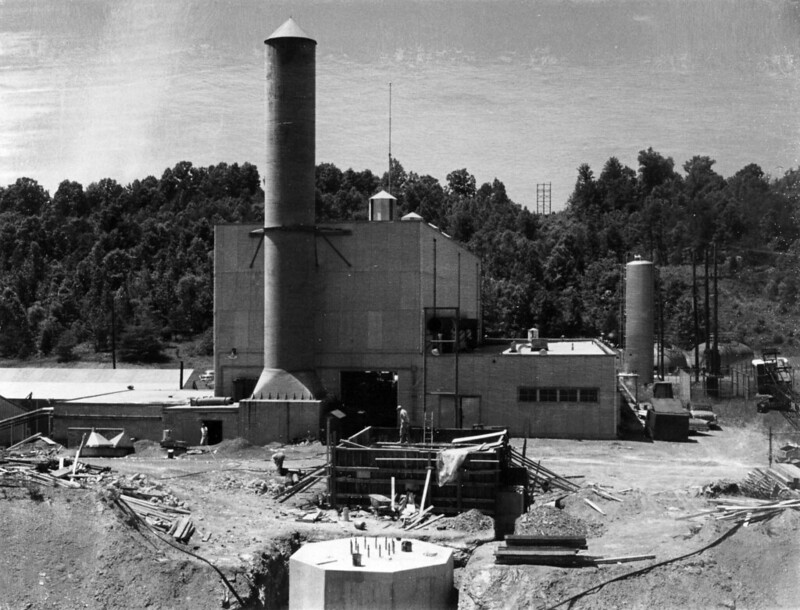
No risk of meltdowns
The perhaps greatest advantage of MSR is that the danger of meltdowns disappears. Emblemsvåg explains: “If a regular light water reactor runs too long, the structural integrity of the fuel assembly worsens due to radiation. Then you can experience some security issues. This means that these reactors can only take out 5-8 percent of their energy potential before the fuel assemblies must be replaced”. In an MSR, you do not have to worry about the structural integrity of the fuel because the fuel is already melted with the salt. “That means you can extract much more energy without having to worry about the structural integrity of the fuel assembly. MSRs thereby become between 25-50 times more efficient than conventional light water reactors”.
They also operate on 7-800 degrees celsius, far below the 1400 degrees boiling point, and the reaction slows down when the heat increases. Should the power be cut, an ice block at the bottom of the reactor melts, which makes the salt run into a different tank and cool down, and the entire reactor stops.
Long-term corrosion?
But the reactor is not perfect. Salt is corrosive, and we do not yet know how the corrosion affects reactors over time. In the 1970s, researchers found a way to circumvent corrosion by using a steel alloy called Hastelloy N. After a ten-week long test of a reactor using Hastelloy N no corrosion was found, but we cannot know how corrosion will affect today’s reactors long-term until they have run for 10-15 years. In the worst case, the reactor vessels and -piping just have to be changed more freqently, which then becomes an economic question.
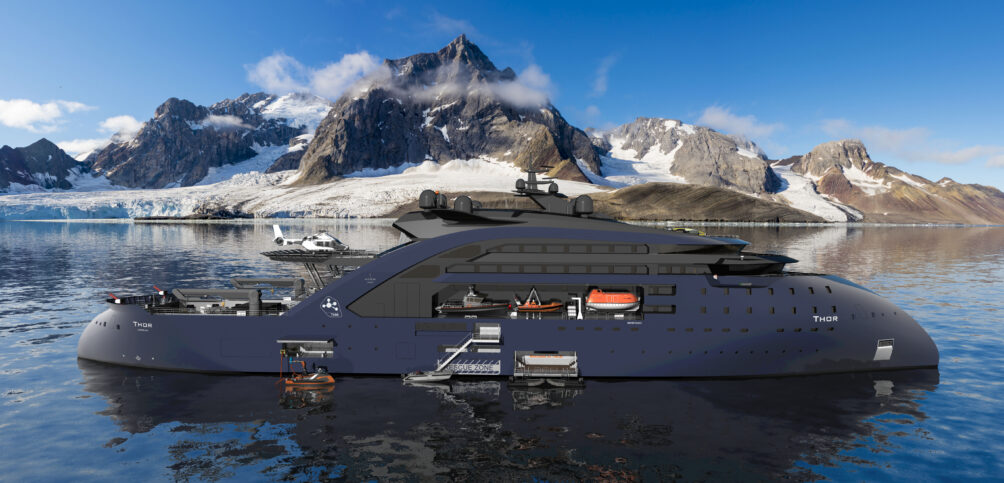
Generation IV: Already up and running
“I think we will have to wait a little bit compared to North-America and China, but there will probably be an operative prototype here in Europe before 2030. When the prototype is made, the next step is to commercialize it, which will take some time, perhaps 3-4 years depending also on regulatory bodies. In Europe, we will probably have molten salt reactors by 2030-2035. China is probably ten years ahead of us, the US at least five years,” says Emblemsvåg.
The first molten salt test-reactor since the 1960s is already up and running in China. If things go according to plan, commercial reactors of about 370 megawatts will be introduced before 2030. In Europe, we might wait a little longer before the first reactors are up and running. One project worth paying attention to is ULSTEIN-THOR. In april 2022, shipping company Ulstein launched the project, in which they work to create battery-run ships charged with thorium-based molten salt reactors. It is too early to say when the project will be due, but the company estimates that the technical development, followed by the approval process of international regulations and classifications, will take 10-15 years, with a goal of commercialisation before 2035.
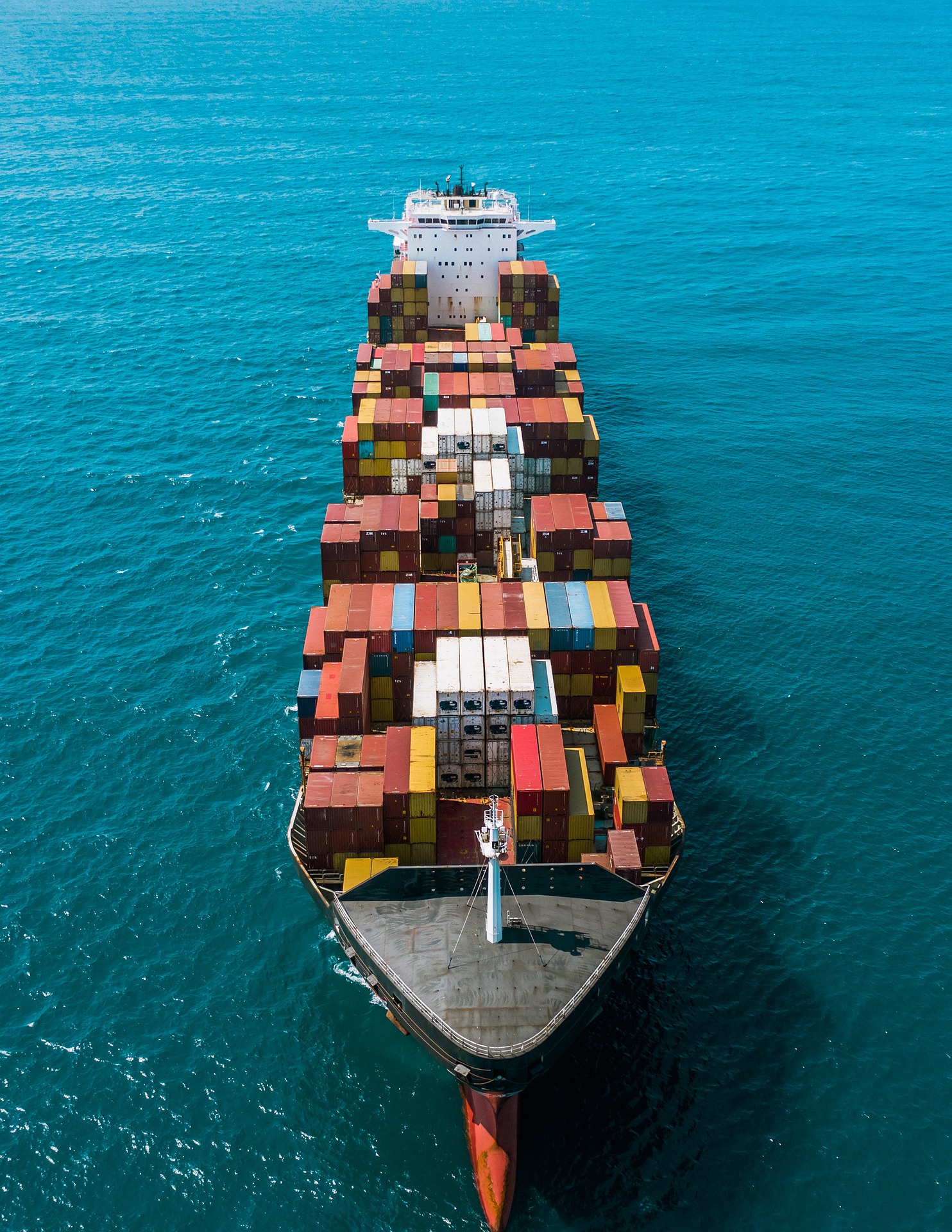
Some alternatives to today’s light water nuclear fission reactors are already up and running. China began operating its first commercial generation IV reactor in December 2021. It is a high-temperature gas-cooled reactor capable of producing about 200 megawatt.
Lead-cooled fast reactors, using molten lead or lead-bismuth eutectic as primary coolants, are also under construction. LeadCold in Sweden aims to have their first prototype in operation by 2027-2028, and first commercial reactor by 2032.
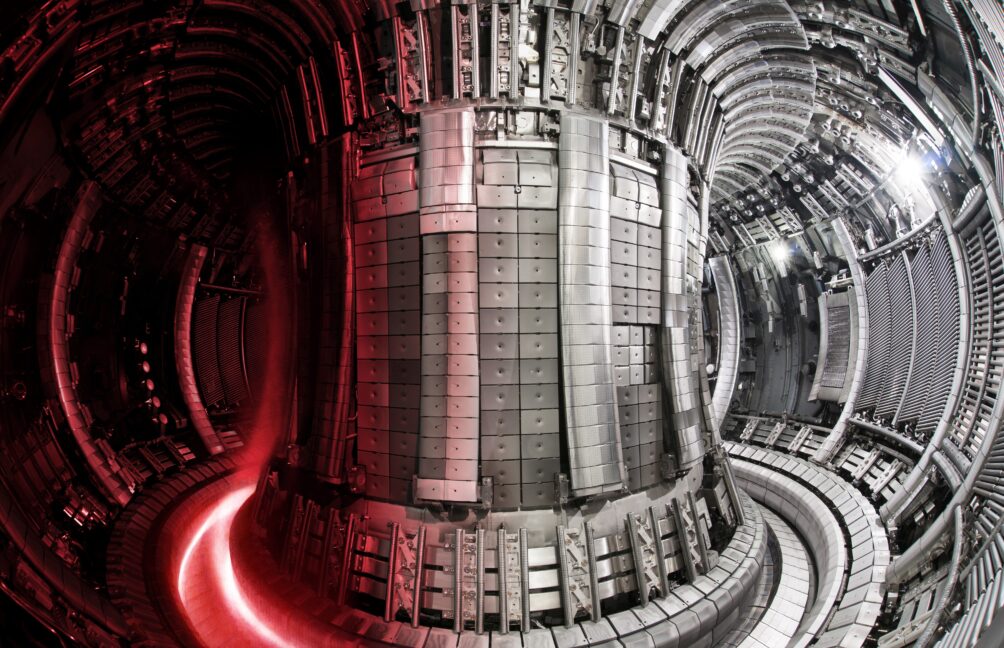
Nuclear fusion energy: the power of the sun
Nuclear fusion is the same type of energy that powers the sun. Two or more nuclei are fused together, and in the process energy is emitted. Just like generation IV reactors, nuclear fusion has several benefits. Besides the abundance of resources, nuclear fusion reactors can be built to be safer than a fission reactor.
Much less radioactive waste is produced. The few radioactive waste that has to be stored has a much lower decay time, and needs to be stored for 100 years as opposed to 10,000 years. Nuclear fusion could also provide much more energy than today’s most powerful commercially operating energy sources. According to nuclear fusion project ITER, “Fusing atoms together in a controlled way releases nearly four million times more energy than a chemical reaction such as the burning of coal, oil or gas and four times as much as nuclear fission reactions (at equal mass).”
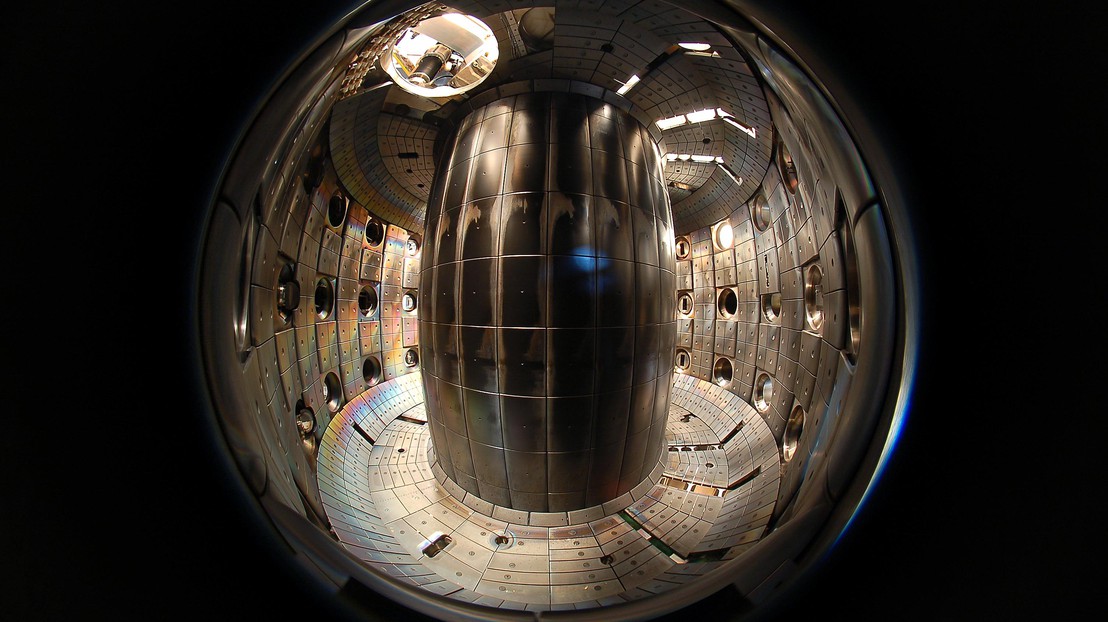
How it works
Inside a fusion reactor, magnetic fields confine a gas consisting of the two hydrogen isotopes deuterium and tritium. The gas is heated up into a state of plasma. In the process, electrons are stripped away from the atom, leaving a charged atomic nucleus and the electrons separated. The nuclei spiral around the magnetic field lines until they bump into each other and form a heavier atom. In this case, the hydrogen atoms form a helium atom. From this, we can form energy.
“When you combine deuterium and tritium they form a helium atom and a high-energy neutron which flies away. Because it is not charged, the neutron is not kept in by the magnetic field. It flies straight out of the plasma and into the walls of the machine”, explains Richard Kembleton, lead researcher of the United Kingdom Atomic Energy Authority (UKAEA), one of several institutions working in collaboration through the consortium EUROfusion. “By putting metal walls, a water cooler, and similar materials in the wall, you can extract the energy from that neutron to produce heat which you can then turn into electricity.”
Afterward, the neutron can be used to create more tritium by reacting to lithium in the wall. This makes the reaction self-sustaining.
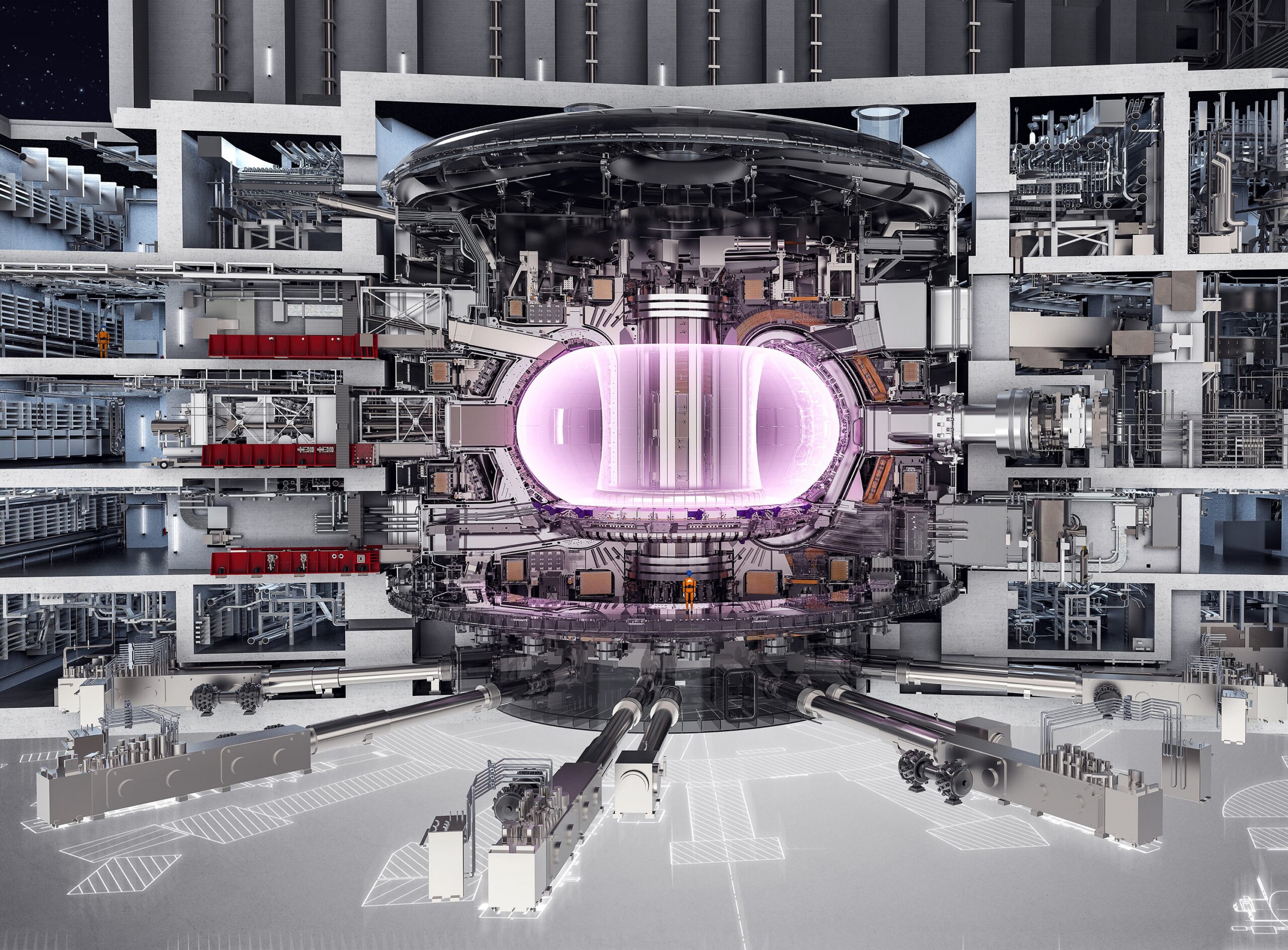
Moving to the technological stage
Have you ever heard the joke about nuclear fusion? It is always 30 years away from being used. Scientists have been researching nuclear fusion for the last 70 years, and so far, the research has focused on the physics behind it. But we are currently in a major transition of nuclear fusion research. We are now moving into a stage of tech development, figuring out how we can build reactors which enables us to control the plasma, find the right materials to build the reactor, and find a way to produce and collect more energy than is needed to run the reactor.
New generators use superconducting magnets for the magnetic field, lithium for the walls, and steel for the actual construction. The inside is made from tungsten, which has a high melting point and is therefore resistant to the heat from the plasma. “On the bottom of the machine you’ve got a device called the diverter, which is where the plasma is designed to touch the machine. That’s an exhaust port. All of the material which doesn’t get burnt comes down to the diverter. It hits the surface and gets cooled down and pumped away,” says Kempleton.
Choosing the right materials
The transition from theoretical research to technological development is an important step towards the dream of using nuclear fusion commercially. We know that creating nuclear fusion on earth is possible, and now we find the materials and develop reactors which allows us to do so.
“One of the problems with the neutrons is that they can turn other atoms into other kinds of radioactive materials. We need to make sure the materials we build the reactor out of don’t produce highly radioactive or long life-time radioactive waste,” says Kempleton. Right now, the promising solution features steel for the reactor design, superconducting magnets, and high-temperature resistant tungsten for the shielding wall inside the reactor. Tungsten has good thermal conductivity, low erosion, and it does not absorb tritium easily, which means it does not easily produce other radioactive materials.
Current projects
The first nuclear fusion test reactors have been in construction for a long time, and some are already in operation. In the UK, JET is already in operation, and is now strong enough to produce the same amount of energy as the reactor requires to run. General fusion and Tokamak Energy both work in the private sector to realise the dream of nuclear fusion energy.
Located in Southern France, scientists from all over the world have joined in to create the world’s largest nuclear fusion reactor, ITER. It is a massive collaboration project between the 27 EU states, Switzerland, the United Kingdom, China, Russia, Japan, South Korea, India, and the United States. The estimated cost of ITER is 20 billion euros. Its goal is to prove that nuclear fusion is a realistic future energy source.
ITER is expected to be completed late December 2025. After that, the reactor will run for ten years as a test. The first commercial reactors can be up and running by the 2050s.
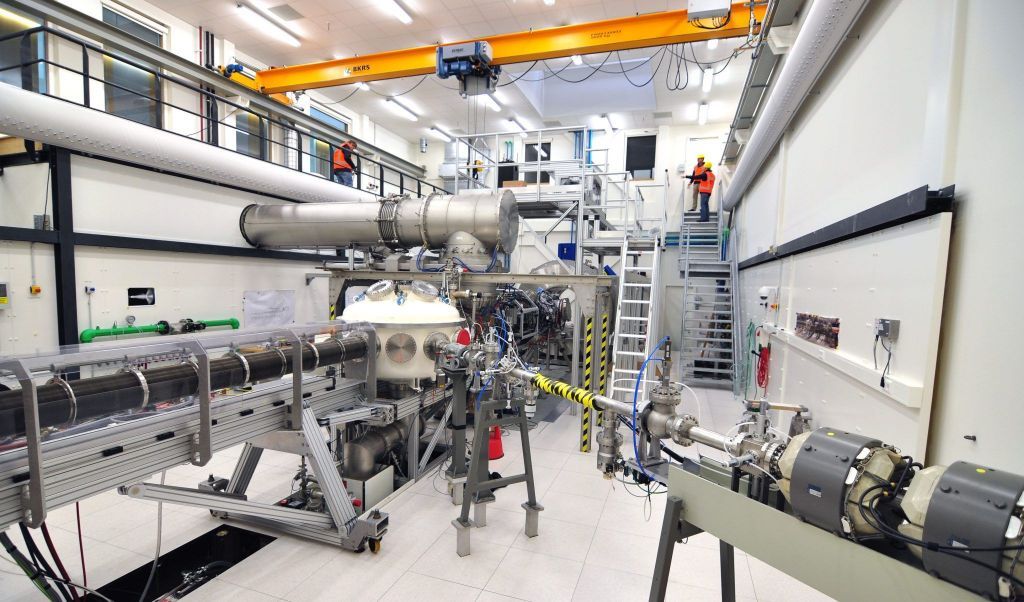
Support us!
Innovation Origins is an independent news platform that has an unconventional revenue model. We are sponsored by companies that support our mission: to spread the story of innovation. Read more.
At Innovation Origins, you can always read our articles for free. We want to keep it that way. Have you enjoyed our articles so much that you want support our mission? Then use the button below: