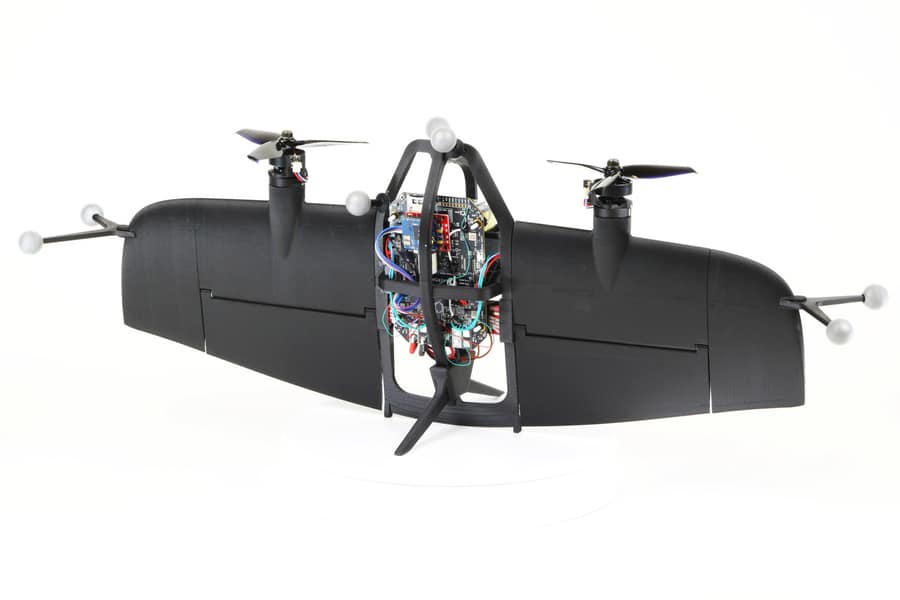
In a pioneering development, MIT researchers have created a novel algorithm for aerobatic trajectory generation in vertical take-off and landing (VTOL) tailsitter flying wing aircraft. The researchers published their findings in IEEE Xplore. Differing significantly from existing fixed-wing trajectory generation methods, this algorithm utilises a realistic six-degree-of-freedom (6-DOF) flight dynamics model, including aerodynamics. This allows for agile manoeuvring, including stunts such as sideways uncoordinated flight and inverted flight. The algorithm’s robust capabilities have been demonstrated through extensive flight experiments, showcasing aerobatic manoeuvres, a time-optimal drone racing trajectory, and an airshow-like aerobatic sequence for three tailsitter aircraft.
- MIT researchers have developed a groundbreaking algorithm for aerobatic trajectory generation in vertical take-off and landing (VTOL) tailsitter flying wing aircraft.
- The algorithm’s efficiency is derived from snap minimization within the differentially flat output space, enhancing computational efficiency.
- This innovative algorithm holds vast potential for applications like search-and-rescue missions, autonomous flights, and parcel delivery.
A new approach to flight dynamics
One of the distinguishing features of this algorithm is its use of a realistic six-degree-of-freedom (6-DOF) flight dynamics model. This model incorporates aerodynamic equations, providing a comprehensive approach to understanding and predicting the behaviour of the aircraft in various flight conditions. Traditional flight dynamics models often simplify the system or use separate models for different flight modes. However, the use of a global dynamics model in this algorithm enables it to cover the entire flight envelope, thereby allowing for aggressive aerobatic manoeuvres under diverse conditions.
The global dynamics model is also instrumental in the algorithm’s ability to exploit the differential flatness property of the tailsitter flying wing’s dynamics. Differential flatness is a property of a system where the system’s outputs can be written as functions of a reduced set of variables, called flat outputs, and a finite number of their derivatives. For the tailsitter aircraft, the position and orientation are the flat outputs. Utilising this property allows for efficient trajectory planning and control of the aircraft.
The power of snap minimisation
Another key aspect of this new algorithm is the implementation of snap minimisation in the differentially flat output space. In the context of drone flight, “snap” refers to the fourth derivative of the position with respect to time, which indicates the change in the “jerk” or the rate of change in acceleration of the drone. Minimising snap essentially smoothens the drone’s trajectory, reducing abrupt changes in motion and making the flight more efficient.
This snap minimisation is performed in the differentially flat output space, a space where the system’s outputs are functions of the flat outputs and their derivatives, but independent of the system’s internal states. This approach allows for efficient computation, as the optimisation problem is reduced to a simpler form compared to the more complex state and control input space. The result is a significant enhancement in the computational efficiency of the algorithm, making it viable for real-time motion planning applications.
Groundbreaking results in the sky
To validate the effectiveness of the algorithm, extensive flight experiments were conducted. These experiments demonstrated the algorithm’s ability to generate aggressive aerobatic manoeuvres, such as loops, rolls, climbing turns, and even synchronised acrobatics. The aircraft successfully tracked these complex trajectories, and the vehicle pose and control inputs closely matched those predicted by the algorithm.
Moreover, these tests included a time-optimal drone racing trajectory, showcasing the algorithm’s potential in competitive drone racing. Racing drones require precise control and the ability to execute rapid manoeuvres, and the successful application of the algorithm in this context underscores its high performance. The algorithm was also demonstrated in an airshow-like aerobatic sequence for three tailsitter aircraft, further highlighting its versatility and the breadth of its applications.
Potential Applications
Given its high performance and versatility, this algorithm holds great promise for numerous applications. Its ability to plan complex trajectories in real-time makes it highly suitable for tasks such as search-and-rescue missions or autonomous flights in dynamic environments. Its speed and efficiency also make it an attractive option for parcel delivery, where the ability to quickly and safely navigate through various conditions is paramount.
By pushing the boundaries of what is possible with VTOL tailsitter flying wing aircraft, this algorithm represents a significant stride in drone technology. Its unique approach to trajectory planning and control, and its proven effectiveness in flight experiments, certainly make it a game-changer in the field of aerobatic drone flight.