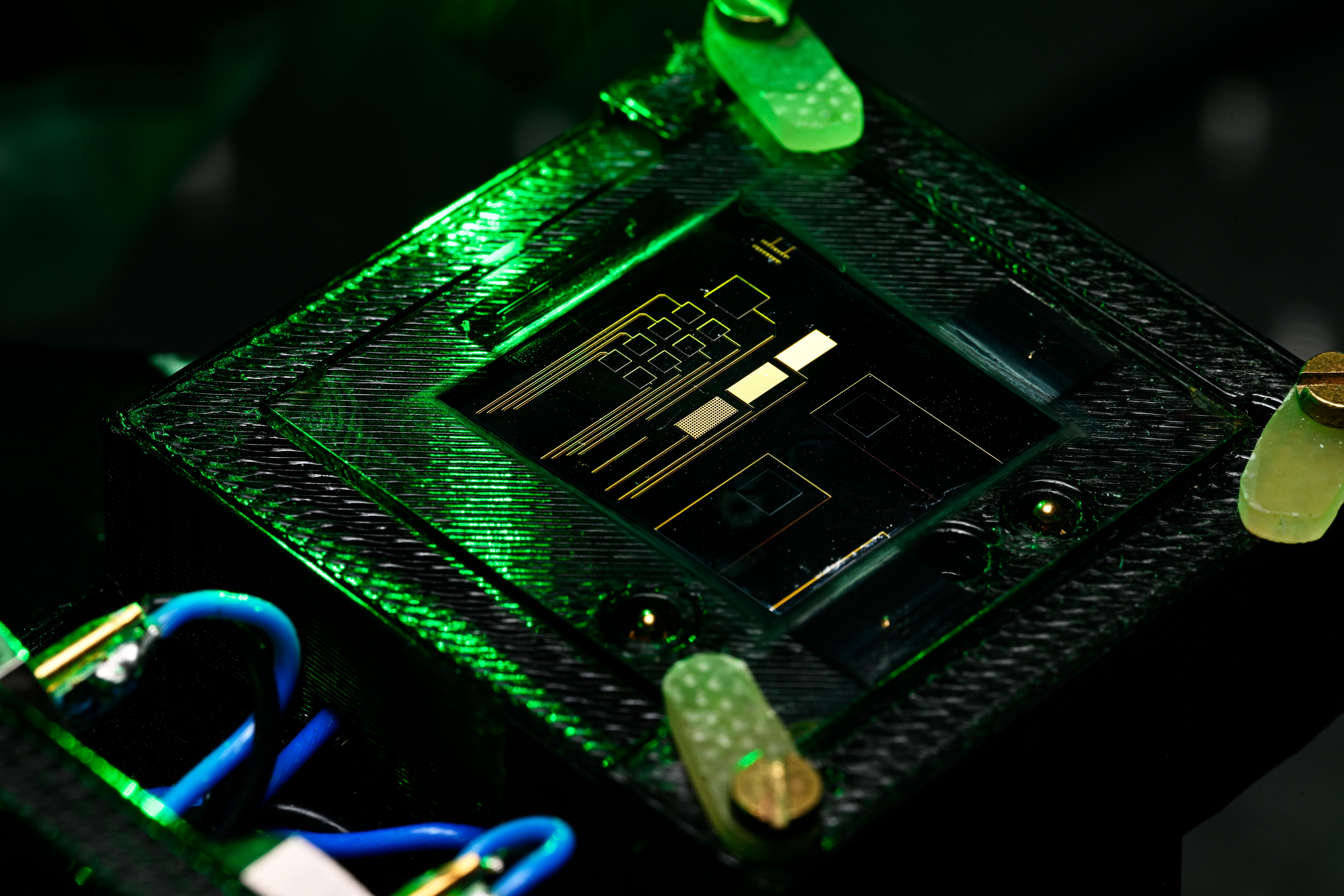
What if your heart rate could be monitored from relatively afar? Would it not be better if hospitalized patients didn’t have loads of vitals-checking machinery attached to their bodies? This is possible with a new light sensor that scientists at TU Eindhoven have developed. However, that remote heart measurement is an application they were not initially looking for. Actually, the researchers were tinkering to make so-called photodiodes work as well as possible. And they succeeded. They even achieved an efficiency of 220 percent. In the so-called “real world”, an energetic efficiency of over 100 percent is, in principle, not possible. However, this is not the case for the quantum realm.
Ph.D. candidate Riccardo Ollearo and Professor of Molecular Materials and Nanosystems René Janssen, who led the study, explain that “quantum efficiency” is the incident photon to converted electron ratio. Here, it is important that the “right type of light” goes through the device and that the right materials are used for the sensor. If these conditions are satisfied, the photodetector can generate current with a two electrons per photon proportion.
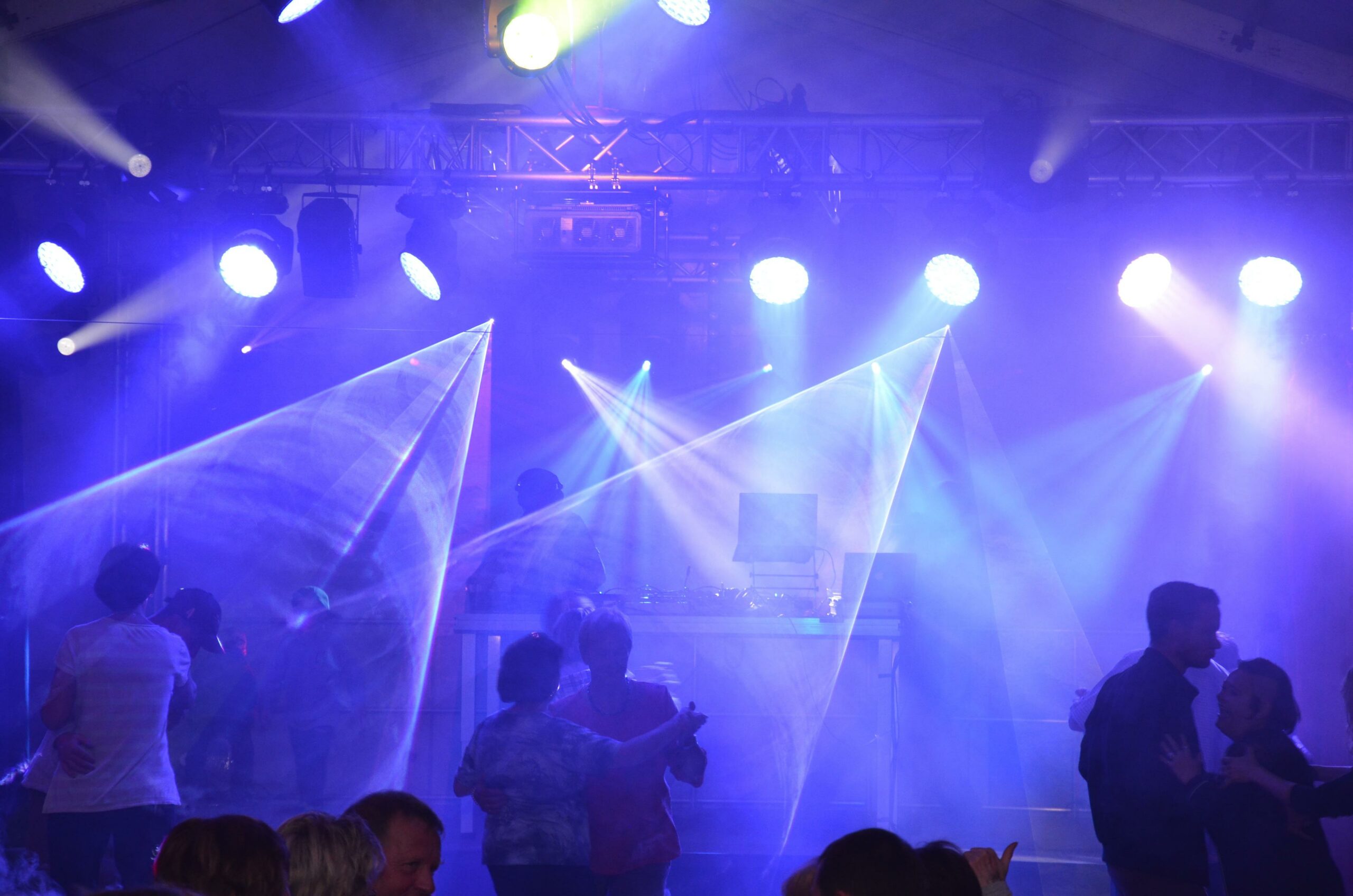
A wide range of real-life applications
This astounding efficiency makes the device really accurate, which has proven helpful in completing various convenient tasks. “This sensor – Ollearo says – could be easily integrated into the medical field. It is cheap and easy to produce, and it could help monitor people in a discreet and non-invasive way. Another example [of its use] – he continues – is when driving a car: it could check your vitals and determine your alertness, constantly checking that you’re not falling asleep”. But these are only two of all the possible applications, which essentially broaden to all of the cases in which distance vitals’ monitoring is necessary. All in all, the sensor could be used in fields concerning medicine, public and private safety and even alongside tech devices like VR goggles.
However, Professor Janssen is cautious when discussing the device’s future and possible usability. “The next steps could involve a study to understand better how this gate between the two layers works. We do not yet fully understand how that works and, hence, we cannot predict if the value that we have now is anywhere close to an upper limit or not. Maybe it possible to enhance the effect. So – he says – before we think further into applications, we need to understand this better. The ultimate goals for work on photodiodes is making them very sensitive, with a large linear dynamic range and, if possible, very fast”.
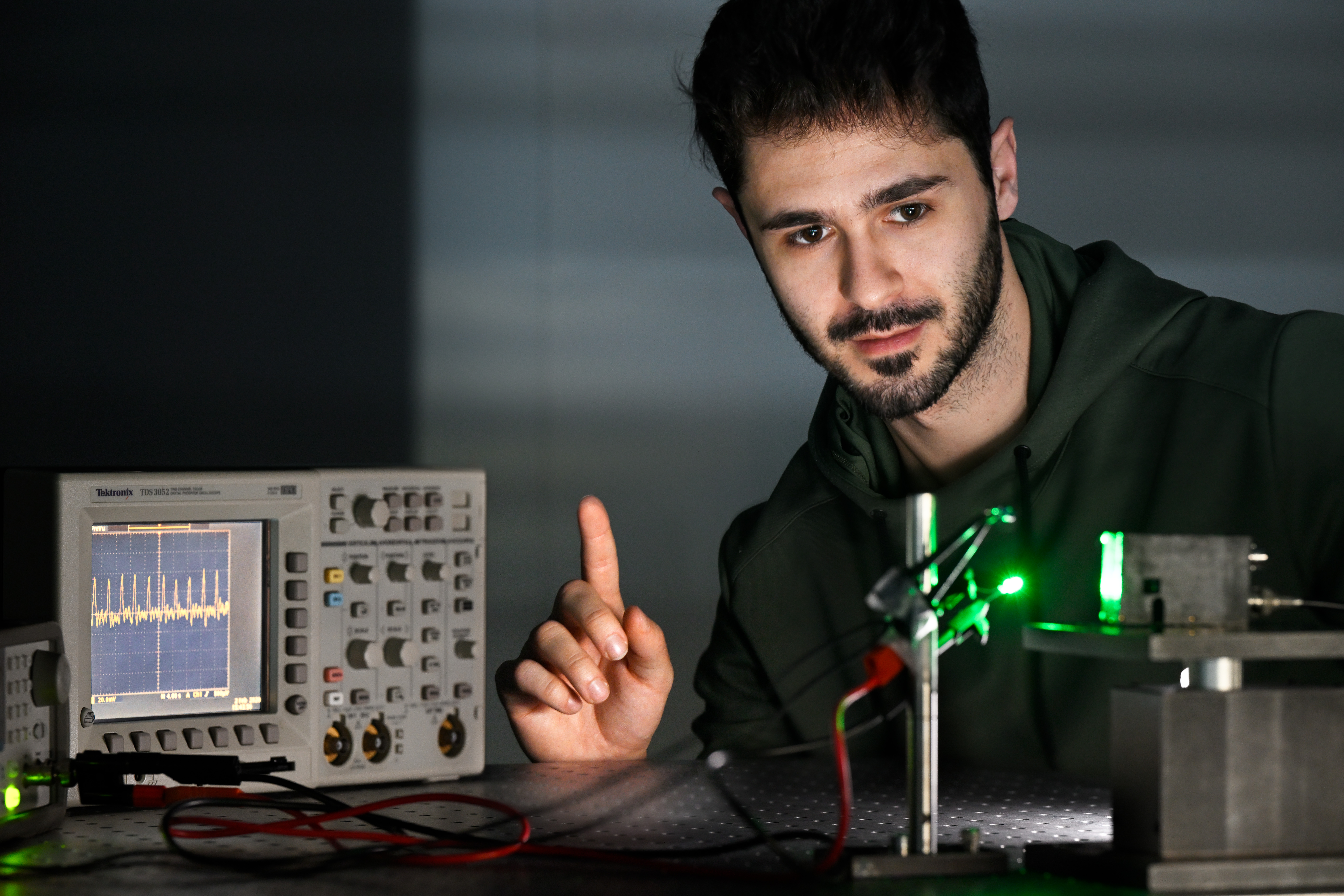
High sensitivity
Indeed, understanding the mechanism behind the light sensor is not immediate. “First of all, – Ollearo begins – there is a big difference to be made between energetic efficiency and what is happening here. The former refers to what goes on in a standard solar cell, where energy from the sun is converted to electricity. This happens because photons excite electrons inside the cell, bringing them from base level to a higher (excited) level, at which charges can move freely and produce energy that can power, for example, a fridge or any appliance. This is not the case for our light sensor, which detects the presence of light, but does not produce energy”.
In fact, the photodetector (or photodiode, in technical language) is operated in a slightly different way than a solar cell, because the goal is not to get energy out of the process, but to extract as much current as possible. Therefore, what matters here is sensitivity, meaning that the sensor, in principle, has to detect even close-to-imperceptible amounts of light going through it.
“Ideally – Ollearo says – you want to have a photon-to-electron efficiency of 1 to 1, or 100 percent. In almost all cases, this is not achievable: some photons are reflected away, others are not absorbed by the material in the cell and, again, others lose their excitement before making it through the material. So out of a 100, you might get a quantum efficiency of 70-80 percent. What we managed to do – Ollearo states – is to get to over 200 percent quantum efficiency”.
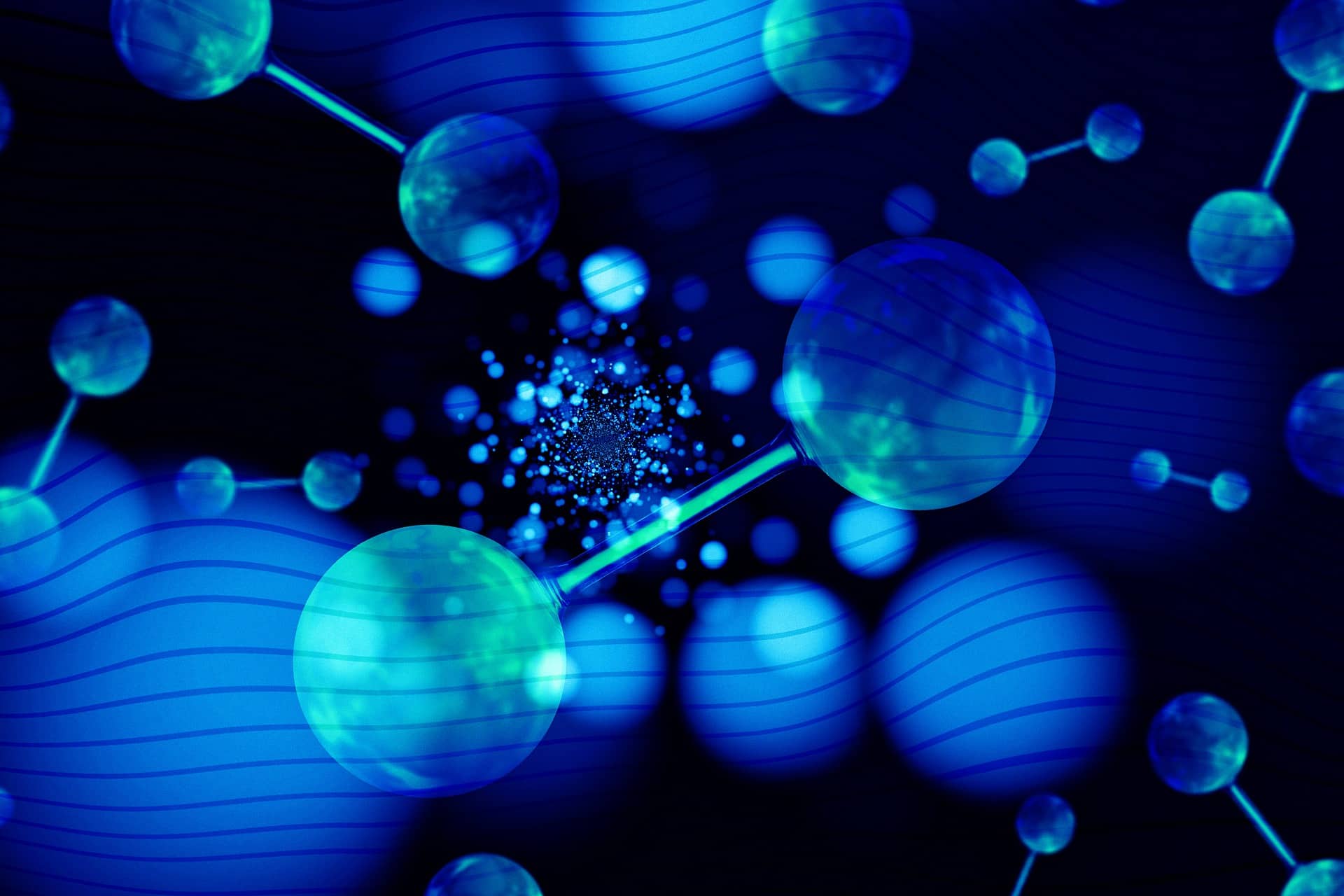
What it needs to work
As mentioned, this has to do with a couple of factors. The first one is the materials Ollearo and his colleagues used: a combination of perovskite and organic materials stacked on top of each other, creating what is technically called an “in a tandem” configuration. The other is the combination of green and infrared light shot through the materials, allowing for the 2 to 1 output. The system works because it utilizes the properties of these two “types” of light, with the green one being characterized by high energy and short wavelength, while the infrared is found at the opposite end of the spectrum, with low energy and longer wavelength.
This combination, where the green light is shot first and the infrared light just after, allows electrons within the aforementioned materials to gain enough excitement to be able to go through the materials’ different layers. This process not only converts photons into electrons efficiently but also “picks up” and brings along electrons that already were left inside the materials and could not jump through material layers on their own, as is the case for those generated by green light.
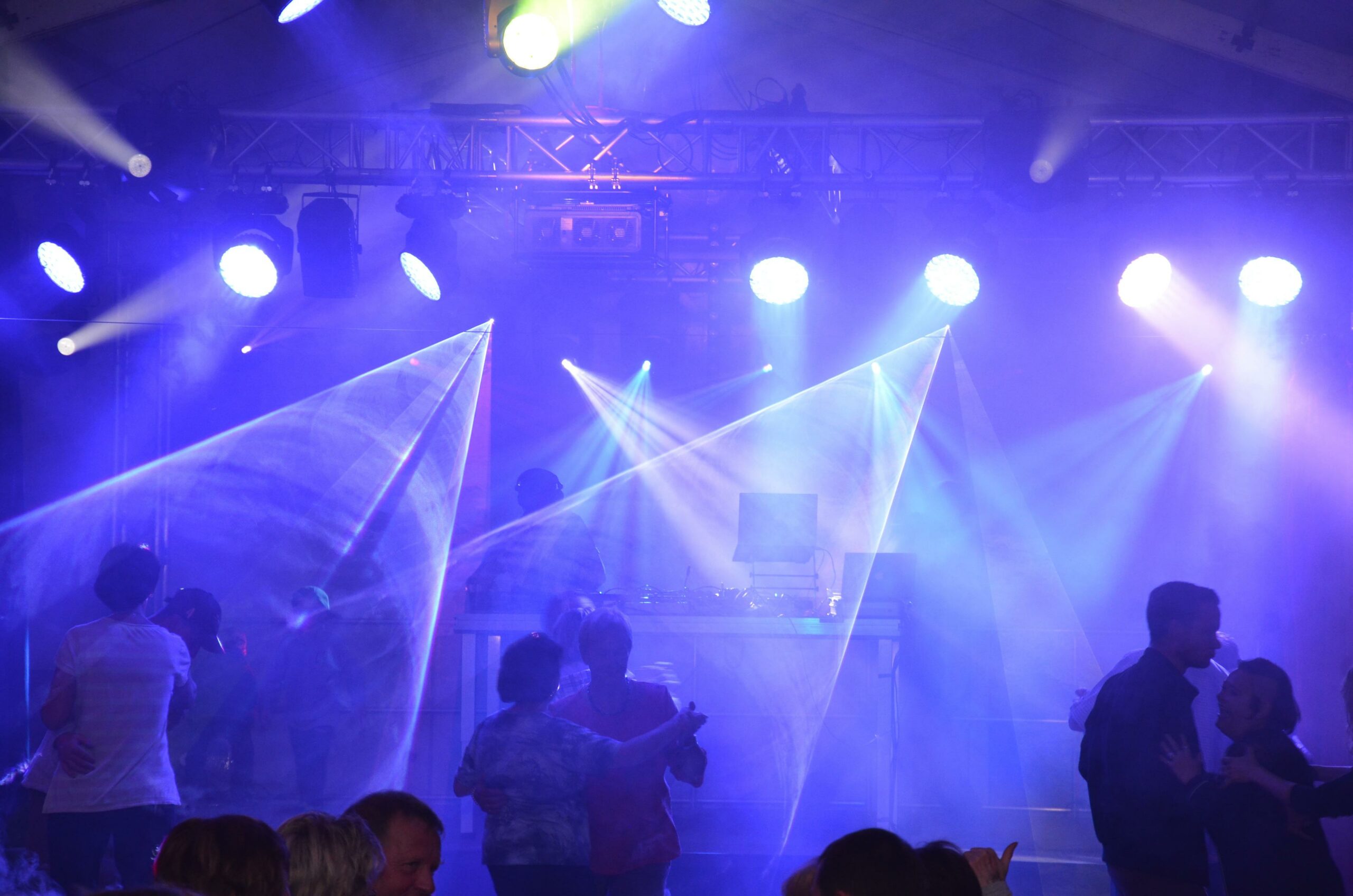