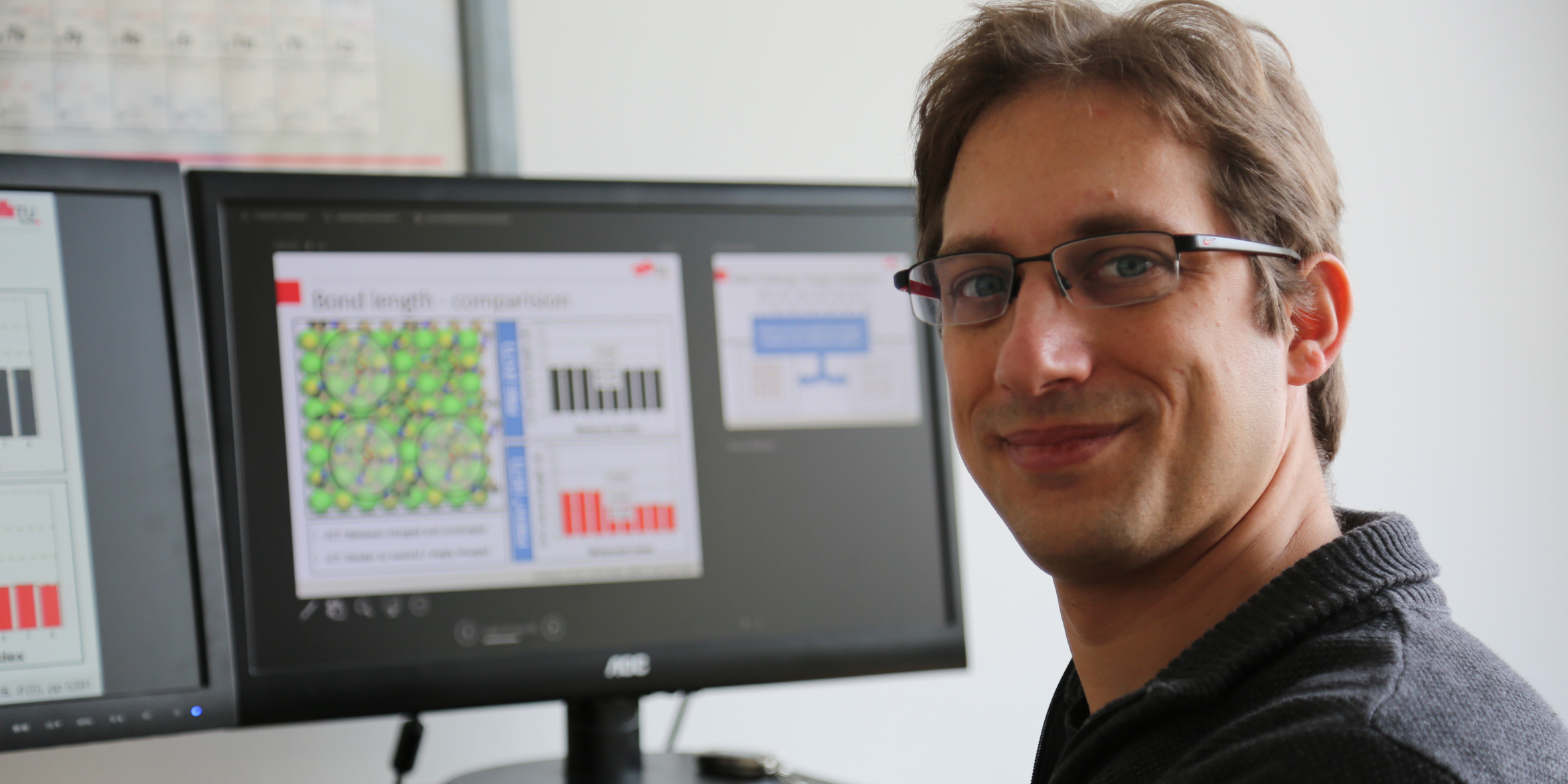
Whether it concerns OLED displays or organic solar cells. Anyone who wants to optimize their operations must take into account how the hybrid materials that are used interact with each other. That is why Oliver Hofmann and his research group at the Institute of Solid State Physics at TU Graz, Austria, have started to research this.
The team has recently focused its attention on the concept of long-distance transfer of charges. A transfer of electrons from one material to another can already take place in a disconnected state. In that case, however, there needs to be more energetically favorable states for the electrons in the adjacent material.
Investigating how deeply electrons interact
So far, scientists have not been able to agree on how far this transfer of electrons in organic material can go. Hence, the first fundamental question posed was: Up until which molecular position does this effect take place? Numerous studies report that this effect is limited to the first layer in the organic-inorganic interfaces. This is the layer where the organic molecules (organic layer) are in direct contact with the metal surface (inorganic layer).
Other studies assume that the effect also extends over greater distances to the second layer or even further. And that is precisely what makes it interesting: “If this is the case, the effect can be used to reduce the electrical resistance of the hybrid material, thus making it more energy-efficient,” states Hofmann, explaining his research interest.
‘We wanted to solve this mystery’
In order to be able to track these organic-inorganic interfaces even more accurately and above all to demonstrate charge transfer over long distances, the researchers examined a copper-tetracyano-ethylene interface (TCNE/Cu(111)). “Because there is particularly strong experimental data here that is indicative of long-distance charges,” Hoffmann explains.
To date, there is no clear theory as to why some systems produce this effect. But Hoffmann and his team wanted to “solve this mystery to establish a basis for making materials with the same property.”
The scientists used two new machine learning methods for their analysis, SAMPLE, and BOSS. By combining both methods, the researchers were able to identify more than two million potential interface structures for TCNE-Cu transfers. On top of that, they were able to predict the behavior of the molecules under a variety of experimental conditions. Surprisingly, the results showed that there is no long-distance charge transfer! Instead, the molecules in the system change their structures.
Molecules that are lying down will stand up at a certain density
If you place a layer of molecules on something, they usually keep their regular order. Moreover, they tend to try to move closer together until, at a certain density, they eventually grow from the first to the second layer.
This is different in the TCNE/Cu(111) system. Here the molecules go from their original horizontal position to an upright position at a certain density, in order to get even closer together. “However, upright molecules have a completely different charge transfer than horizontal molecules,” explains Hofmann. “The structural change is difficult to detect in experiments, but the measurement results are comparable to those of the charge transfer over long distances.” Consequently, the research contradicts the hypothesis of long-distance charge transfer. In the future, these new findings can be used directly in the development of new materials to improve the efficiency of electronic components.
Using machine learning provides insight
The use of the combined SAMPLE and BOSS machine learning methods is helping to assist future experiments in materials development in such a way that any similar misinterpretations are avoided. By going deeper into the physical processes, the new methods help avoid designing materials that aim for an effect that does not exist in this form. Hofmann emphasizes the advantage of the new method: “The two methods will make it possible to simulate millions of different structures in the future.”
The researchers at TU Graz recently published details of the study in Advanced Science.